Eelgrass is Great,
but Shellfish Aquaculture is Better
Presented by Robert B. Rheault on January 11, 2001 at Marine Aquaculture and the Environment: A Meeting for Stakeholders in the Northeast
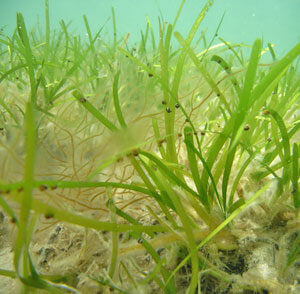
Photo by C. Pickerell
There are many cases where aquaculture can have profound positive impacts on the environment. Any time you place a structure in the water it establishes a new habitat, acts as a fish-aggregating device and supports a myriad of new recruits. Local diversity may be affected, productivity can be enhanced and many other benefits can be observed. In oligotrophic waters moderate enrichment by fish food or feces will cause an increase in productivity. Preclusion of dragging activity by the physical presence of aquaculture gear has proven to benefit local ecosystems. Even periodic dredging of bottom-cultured shellfish can stimulate and enhance certain aspects of the ecosystem. Perhaps these alterations are not "natural," but we must begin to accept that there are no longer any ecosystems on earth that remain unaltered by human activities, and we need to learn to evaluate the impacts of those activities in this light.
In response to federal regulations established by the National Marine Fisheries Service, states are now required to "preserve, protect and where possible, restore submerged aquatic vegetation" (SAV), especially eelgrass. Eelgrass serves a number of important ecological functions in the marine environment. The plants take up nitrogen from the water column; the rhizomes stabilize the sediment and promote denitrification; the grasses reduce turbulence, allowing particles to settle out, which increases water clarity; and the fronds support a wide diversity of marine life and provide excellent habitat for a number of important species of fish and shellfish.
In recent years eelgrass beds have been declining up and down the Atlantic coast. The causes of these declines are well documented (Kemp et al., 1983; Twilley et al., 1985; Short and Burdick, 1996). Excessive nitrogen loading in coastal waters from anthropogenic activities leads to increased phytoplankton blooms, which reduce light penetration. The bottom-dwelling plants suffer from inadequate light, and like any vascular plant, eelgrass responds to high nitrogen and light limitation by elongating and becoming more fragile. The result has been dramatic, especially in coastal estuaries where nitrogen fluxes have increased several-fold due to unchecked upland development. Important bay scallop habitat has been eliminated and coastwide harvests of this valuable species and many others continue to be impacted.
Since the federal laws protecting SAV came out, several aquaculture operations have come under fire. Large clam farms in Virginia and oyster farms in Washington State have come under heavy regulatory pressure to cease or move because of perceived interference with eelgrass. Fortunately, these farms, which support hundreds of jobs and generate millions of dollars worth of shellfish, have been allowed to continue working while they try to prove that they are not deleterious to the environment.
In 1998 the Rhode Island Coastal Resources Management Council enacted new regulations to protect eelgrass beds. Policies suggest that areas with existing or historical eelgrass should be protected from aquaculture development. I love eelgrass as much as the next guy, but I object to the policies that would displace shellfish aquaculture in favor of eelgrass.
If we really want to save the eelgrass we need to address the issues of over development in the watershed. If we don't stem the nitrogen sources (primarily lawn fertilizer and septic systems) it will not matter how hard we try to protect the eelgrass from other disturbances. Restoration efforts will be futile as long as the habitat remains unfit for eelgrass recovery.
Shellfish aquaculture can give us all of the same wonderful ecological benefits of eelgrass and more. In fact, it would appear that the seagrasses now returning to oyster beds in Washington and to clam beds in Virginia are doing so because shellfish culture activity makes it possible. Shellfish are active filter feeders, cleansing millions of gallons of water each day, improving water clarity and light penetration, making it possible for the eelgrass to flourish in grounds that have not supported seagrasses for decades. Without shellfish aquaculture the mud and sand in these beds are too unstable to support the rhizomes of these aquatic plants. In these cases the mere presence of culture gear and shell provides the necessary benthic stability for the SAVs to tolerate storm events.
No one questions that seagrass beds provide excellent habitat for juvenile fish, crustaceans and shellfish. In comparison to unvegetated areas, eelgrass beds have higher species diversity indices, and higher populations of a wide variety of commercially valuable fish and forage species. But the fact is that almost any structure in the marine environment stimulates similar increases in diversity and abundance. Aquaculture gear in particular provides prime habitat, attracting huge numbers of juvenile fish and crustaceans that aggregate to feed on the fouling organisms and to seek refuge in what is essentially an artificial reef.
I have asked a graduate student to document these facts on my shellfish cages in Narragansett, R.I. I work in a coastal salt pond that has extensive development along its shores. Nitrogen levels are elevated and phytoplankton concentrations average about ten times those just outside the pond. Eutrophication is severe in this rich, depositional environment, and hypoxic and anoxic conditions are a growing concern.
When I applied for my 2.3-acre lease seven years ago the bottom was characterized as dead anoxic mud and shellfish surveys revealed only a handful of clams in the site. I now have over 400 cages on this site and I have observed a dramatic increase in the numbers of a variety of organisms. Fish and crustaceans are attracted by the fouling organisms that grow on the cages and juveniles seek refuge in the interstices. We see dozens of juvenile and adult fish in every cage we pull up.
In the spring we see juvenile lobsters in nearly every cage. Commercially important species such as tuatog, black sea bass, eel, scup, winter flounder, and blue crabs are now common on our lease. The lease has become one of the most popular recreational fishing spots on the pond.
The cages also attract numerous important forage species such as cunner, tomcod, rock eel, minnows, stickleback, and a wide variety of crabs. These species in turn attract a seasonal procession of transient predators, including striped bass, adult winter flounder and shad to name just a few.
A graduate student from URI is in the process of documenting the increased productivity, species diversity and species richness that we have observed since we started working here. I suspect that these indices will compare quite favorably with data collected in nearby eelgrass beds and unvegetated bottom.
Over the same time period that we have observed these changes on our lease, the eelgrass beds that used to grow a few hundred yards to the south have retreated to nearly a mile away, nearer to the opening of the pond where ocean water dilutes the nitrogen and phytoplankton blooms that persist further up the pond. As watershed development continues unabated, anthropogenic nitrogen inputs are increasing. Phytoplankton blooms are getting richer, light penetration is diminishing and anoxic events are becoming more pronounced. These impacts are well documented (Twilley et al., 1985; Short 1996).
Seagrasses have been credited with removing particles and nitrogen from the water column, but when the grasses die back in the fall any nitrogen assimilated by the grasses is released back to the environment as the grass decays. Contrast that with shellfish. Dr. Michael Rice (in press) has estimated that for every kilo (wet weight) of harvested shellfish meat, 16.8 grams of nitrogen are permanently removed from the ecosystem and shipped off to Manhattan. The harvest from my small farm in Point Judith Pond compensates for the nitrogen inputs of about 133 people in the watershed.
The active filtering activity of shellfish is many times more effective in clearing particles from the water column than the passive settlement of particles seen in seagrass beds. Oysters, in particular, are extremely effective at clearing particles as small as 1 micron, which have a very slow natural sedimentation rate (Mohlenberg, F. and H.U. Riisgard. 1979; Haven and Morales-Alamo, 1970). The active filtration by a few million oysters can clear hundreds of millions of gallons of water daily (Cloern, 1982; Officer et al., 1982). This activity improves water quality and enhances light penetration, which should (if there are enough shellfish) help the SAVs to recover.
A substantial portion of the particulate organic matter that is filtered from the water column is deposited on the bottom as feces and pseudofeces where it is made available to deposit-feeding invertebrates (Reid, 1981). The organic enrichment of sediments also enhances denitrification activity (bacterial reduction to nitrogen gas and N2O) serving as yet another important nitrogen sink for environments suffering from excessive anthropogenic enrichment (Seitzinger et al., 1984; Seitzinger and Nixon 1985, Doering et al. 1987).
Instead of denying permits for aquaculture in areas where eelgrass is (or was at one time) present, regulators should be encouraging shellfish aquaculture, since it can perform all of the desired ecological functions as well as, or better than, the adjacent eelgrass beds. While these artificially enhanced shellfish populations may not be "natural" in a strict sense, they preserve and enhance the ability of the ecosystem to remain productive. The shellfish remove nitrogen from the system and the increased diversity associated with these structures enhances the stability and resiliency of the entire ecosystem.
Dredging - Bottom Culture
Many shellfish farmers do not use cages to grow shellfish. Instead they lay the shellfish on natural bottom and use rakes or dredges to harvest them. While the disturbance of dredging or raking can be severe, there is substantial evidence that in most environments recovery is rapid (DeAlteris, 2000) and the impact on some species is clearly beneficial (Rask 1987, Peterson et al. 1987).
While dredging no doubt kills many crustaceans and benthic inhabitants, there is strong evidence on oyster beds in Connecticut suggesting that the abundance and survival of juvenile lobster is enhanced by the presence of shell - even though they are regularly dredged. It is well documented that dredging removes the fine sediments, which enhances the larval settlement of many bivalve species. Disturbed sediment often stimulates huge dense sets of shellfish (Woodin 1976).
At least in aquaculture sites, the harvest areas are restricted, and the impacts are localized. The oyster grounds in Connecticut have been dredged for over 100 years. These ecosystems may not be considered "natural," but it is not hard to make the argument that they still provide excellent habitat for a wide variety of species and that the benefits in terms of productivity are clear. It would be interesting to assess the diversity in these grounds in comparison to nearby undredged bottom where silt has covered the shell and the bottom is without features. While neither ecosystem looks like it did 400 years ago, each supports a rich community of organisms.
References
Cloern, J.E. 1982. Does the benthos control phytoplankton biomass in South San Francisco Bay? Marine Ecology Progress Series 9:191-202.
Doering, P.H., J.R. Kelly, C.A. Oviatt, and T. Sowers. 1987. Effect of the hard clam Mercenaria mercenaria on benthic fluxes of inorganic nutrients and gasses. Marine Biology 94:377-383.
Haven, D. and R. Morales-Alamo. 1970. Filtration of particles from suspension by the American oyster, Crassostrea virginica. Biological Bulletin 139:248-264.
Kelly, J.R. and S.W. Nixon. 1984. Experimental studies on the effect of organic deposition on the metabolism of a coastal marine bottom community. Marine Ecology Progress Series 17:157-169.
Kemp, W.M., W.R. Boynton, R.R. Twilley, J.C. Stevenson, and L.G. Ward. 1983. Decline of submerged vascular plants in upper Chesapeake Bay: Summary of results concerning possible causes. Marine Technical Society Journal 17:78-89.
Mohlenberg, F. and H.U. Riisgard. 1979. Filtration rate, using a new indirect technique, in thirteen species of suspension feeding bivalves. Marine Biology 54:143-147.
Newell, R.I.E. 1988. Ecological changes in Chesapeake Bay: Are they the result of overharvesting the American oyster, Crassostrea virginica. Pp. 536-546. In: Understanding the Estuary: Advances in Chesapeake Bay Research. Proceedings of a Conference. Publication no. 29, Chesapeake Research Consortium, Baltimore, MD.
Nixon, S.W., S.L. Granger, and B.L. Nowicki. 1995. An assessment of the annual mass balance of carbon, nitrogen and phosphorus in Narragansett Bay. Biogeochemistry 31:15-61.
Officer, C.B., T.J. Smayda and R. Mann. 1982. Benthic filter feeding: A natural eutrophication control. Marine Ecology Progress Series 9:203-210.
Officer, C.B., R.B Biggs, J.L. Taft, L.E. Cronin, M.A. Tyler, and W.R. Boynton. 1984. Chesapeake Bay anoxia: Origin, development, and significance. Science 223(4631):22-26.
Rice, M.R., In Press, Filter Feeding to Control Eutrophication, Environment Cape Cod.
Rice, M.A. 1999. Control of eutrophication by bivalves: Filtration of particulates and removal of nitrogen through harvest of rapidly growing stocks. Journal of Shellfish Research 18(1):275.
Rice, M.A., A. Valliere, M. Gibson and A. Ganz. 2000. Ecological significance of the Providence River quahogs: Population filtration. Journal of Shellfish Research 19(1):580.
Seitzinger, S.P. and S.W. Nixon. 1985. Eutrophication and the rate of denitrification and N2O production in coastal marine sediments. Limnology and Oceanography 30(6):1332-1339.
Seitzinger, S.P., S.W. Nixon, and M.E.Q. Pilson. 1984. Denitrification and nitrous oxide production in a coastal marine ecosystem. Limnology and Oceanography 29(1):73-83.
Short, F.T. and D.M. Burdick. 1996. Quantifying eelgrass habitat loss in relation to housing development and nitrogen loading. Estuaries 19:730-739.
Twilley, R.R., W.M. Kemp, K.W. Staver, J.C. Stevenson, and W.R. Boynton. 1985. Nutrient enrichment of estuarine submerged vascular plant communities. 1. Algal growth and effects on production of plants and associated communities. Marine Ecology Progress Series 23:179-191.